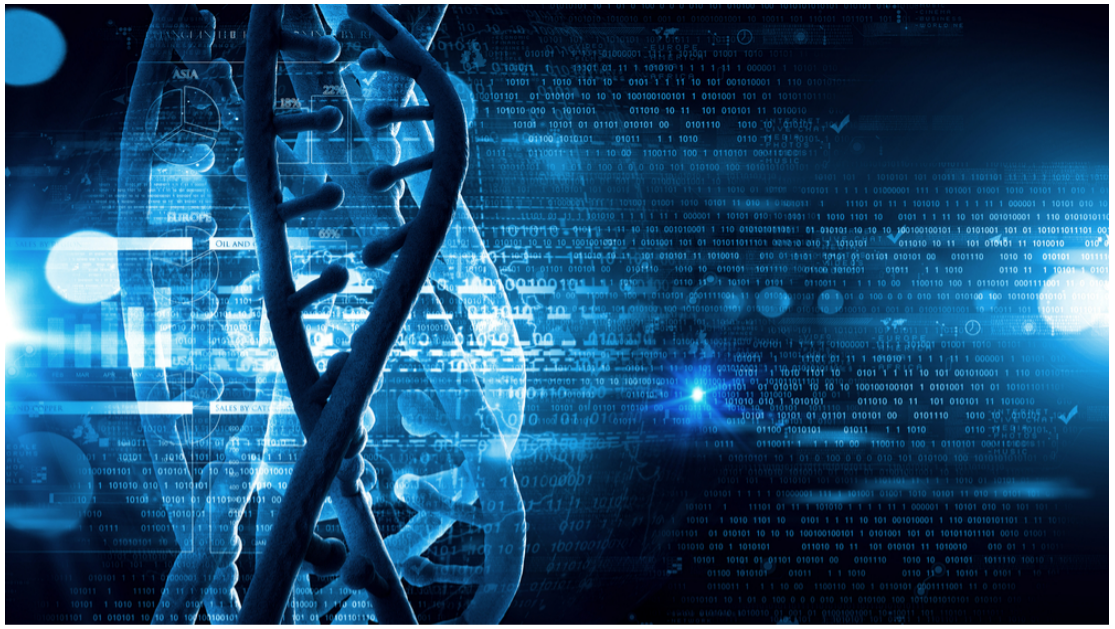
High-throughput RNA-sequencing (RNA-seq) is the use of next generation sequencing technology to explore the transcriptome of a genome. It allows researchers to characterize and compare gene expression profiles of biological samples. RNA-seq is now one of the most common tools in molecular biology.
In this article, we discover the RNA-seq technique, how it works, and how new RNA-seq technologies are driving the ‘ultra-high-throughput’ era of genomics research.
The RNA-seq technique
RNA-seq was developed over ten years ago and has dramatically accelerated transcriptomic research (Lister et al., 2008; Stark, Grzelak and Hadfield, 2019). It has provided new insight into the contribution of gene expression in every area of biology, from normal development to disease contexts.
Researchers commonly use RNA-seq to detect differential gene expression between experimental conditions, diseases or other biological samples. These comparative analyses identify key candidate genes driving diverse processes.
Broader applications of RNA-seq technology extend to mRNA splicing, non-coding RNAs and small RNAs.
Recent technological and computational advances now enable methods such as single-cell RNA-seq, long-read sequencing and ultra-high-throughput RNA-seq techniques such as MERCURIUS™ Bulk RNA-Barcoding and Sequencing (BRB-seq) from Alithea Genomics (Alpern et al., 2019).
How high-throughput RNA-seq works
Classical high-throughput RNA-seq methods usually rely on short-reads and often use Illumina next generation sequencing technologies (Lister et al., 2008).
Firstly, RNA-seq begins with extraction of RNA from biological samples. This is followed by enrichment for mRNA molecules. The enriched mRNA is reverse transcribed into complementary DNA (cDNA). cDNA is then fragmented and adapters are added to the cDNA molecules to prepare a library which is amplified before sequencing.
Illumina sequencing machines are high-throughput because they use ‘flow cells’ to immobilize millions of cDNA molecules simultaneously. To read the bases of each cDNA, the machines image fluorescently labeled reversible terminators as each dNTP is added. These are then cleaved to incorporate the next base. This provides a rapid and accurate read-out of the transcriptome.
After sequencing, computational techniques align the many millions of reads per sample to a reference genome. Following this alignment, researchers can use bioinformatic tools to quantify levels of gene expression. Statistical analyses can then uncover key gene expression differences in comparative studies such as disease versus non-disease samples.
High-throughput RNA-seq technologies now also allow extensive multiplexing. This means many samples can be pooled and sequenced at the same time. One trade-off is that sequencing depth per sample is reduced when more samples are multiplexed in traditional RNA-seq techniques.
Novel ultra-high-throughput RNA-seq technologies
New RNA-seq techniques now allow more samples to be multiplexed than ever before, with no loss in data quality compared to standard RNA-seq techniques at the same read depth (Alpern et al., 2019). Technologies such as MERCURIUS™ BRB-seq from Alithea Genomics use a strategy from single cell RNA-seq.
A sample barcode and unique molecular identifier (UMI) is added to the 3’ end of each mRNA molecule in a sample. This barcode allows researchers to pool thousands of samples and identify each sample and PCR duplicates during data analysis after sequencing.
A lower read depth per sample achieves similar quality data as traditional RNA-seq techniques as only the 3’ end of mRNA is sequenced. Therefore, MERCURIUS™ BRB-seq results in accurate and highly cost-effective ultra-high-throughput RNA-seq experiments at scale.
Contact us at info@alitheagenomics.com to see how MERCURIUS™ BRB-seq can help in your next RNA-seq study.
References
Alpern, D., Gardeux, V., Russeil, J., Mangeat, B., Meireles-Filho, A.C., Breysse, R., Hacker, D. and Deplancke, B., 2019. BRB-seq: ultra-affordable high-throughput transcriptomics enabled by bulk RNA barcoding and sequencing. Genome biology, 20(1), pp.1-15.
Lister, R., O'Malley, R.C., Tonti-Filippini, J., Gregory, B.D., Berry, C.C., Millar, A.H. and Ecker, J.R., 2008. Highly integrated single-base resolution maps of the epigenome in Arabidopsis. Cell, 133(3), pp.523-536.
Stark, R., Grzelak, M. and Hadfield, J., 2019. RNA sequencing: the teenage years. Nature Reviews Genetics, 20(11), pp.631-656.